With hundreds of therapies in development and clinical trial phases, gene therapies are becoming a booming segment. As these products advance to Phase III and into commercialisation, global demand for gene therapy vectors is expected to rise exponentially.1
Existing viral vector manufacturing methods cannot sufficiently support the industrialisation of gene therapies, driving demand for new production technologies.2,3 Most notably, current cell culture technologies face several limitations in terms of scalability, production capacity, and capital and operational costs.
Traditional flatware technologies based on “scaled-out” laboratory principles such as plastic roller bottles and multistack culture vessels are labour-intensive, low capacity and require levels of capital expenditure.
Bioreactor-based production offers scale-up capabilities up to several thousand litres, either for suspension cells or anchorage-dependent cells grown using microcarriers.
However, a significant barrier to industrialisation is the inevitable high infrastructure and equipment investments, especially as these need to be made at a clinical stage when the risk of drug candidate failure is often relatively high.
Single-use, packed-bed bioreactors have emerged as high-density, scalable platforms for viral production.
Yet, these technologies still require optimisation in terms of process monitoring and control, reproducibility and usability. The packed strips and disks are not uniform and can lead to non-homogenous cell distribution within the bed, which reduces titres and limits batch-to-batch reproducibility.4 This can even make it challenging to reach the production capacity required for commercial production.
An intense solution
Univercells’ approach to overcoming these hurdles was driven by the concepts of process intensification and chaining for low-footprint, cost-effective viral production. The resulting scale-X fixed-bed bioreactor portfolio supports the enhanced upstream processing of viral products from early development to large-scale commercial production.
Based on a novel process design, the single-use scale-X bioreactors deliver high cell densities and virus titres. The interior features a compact and structured fixed-bed with alternate layers of custom-treated non-woven fabric and spacing material assembled in a spiral-wound structure. This design provides a large surface area for cell culture in a small volume to ensure rapid and homogenous cell entrapment, media and nutrients availability.
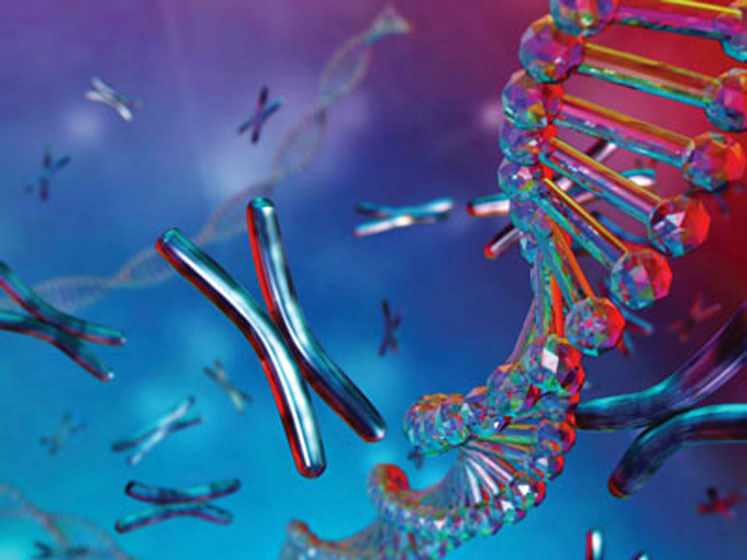
By creating an homogenous environment, reliable cell culture and viral production can be achieved for both adherent cells that will spread and grow on the fibres and non-anchorage dependent cell lines that will be trapped and grow into the structure of fixed-bed.
The single-use scale-X bioreactor is chained with inline product concentration to yield a low-volume, high-titre harvest for simplified and downsized purification operations. Automated sensors and pumps for media addition and parameter adjustments ensure consistent operation with minimal manual interventions — and fixed-bed sampling ports permit cell growth monitoring.
Benefiting from a very low footprint, the scale-X system can be contained in biosafety cabinets or isolators and is fully automated for increased reliability and reproducibility.
Scalability in application
The scale-X family provides seamless scalability from early process development to commercial manufacturing. Scaling-up is based on the principle of constant linear velocity by increasing the surface area available for growth while delivering similar fluid conditions at all scales. This scalability by design ensures predictable cell growth and product behaviours.
The portfolio of bioreactors is suited for process development (scale-X hydro = 2.4 m2), pilot scale and early clinical production (scale-X carbo = 10–30 m2) up to large-scale industrial production when integrated into the NevoLine biomanufacturing platform (scale-X nitro = 200–600 m2).
The design intensifies the process in a drastically decreased footprint. For instance, the scale-X carbo bioreactor with 10–30 m² of surface area is equivalent to 120–360 roller bottles or 16–48 ten-layer multitray cell culture dishes in a total vessel volume of 1.6–3.2 L.
Industrialising gene therapies
Through scalability by design, the scale-X bioreactor portfolio streamlines the transition from process development to clinical trials and commercial production. From a cost perspective, users can stagger investment during the development and clinical validation phases by adapting the scale to the volume required in each phase of the process.
With an intensified upstream process, equipment size, capital and operating costs associated with cell culture and viral production are drastically reduced. Most importantly, the existing bottleneck in the production of viral vectors is eliminated, unlocking the potential of gene therapies and making the market accessible to all types of manufacturers.
Regardless of needs, the optimal solution is available for a range of adherent and suspension cell lines for high-capacity and cost-effective viral vector production necessary for developing and commercialising life-saving novel gene therapies.
References
- N. Faust, et al., “Addressing the Challenges of Commercial-Scale Viral Vector Production,” Cell and Gene Therapy Insights 4, 31–36 (2018).
- J. Yang, et al., “Large-Scale Microcarrier Culture of HEK293T Cells and Vero Cells in Single-Use Bioreactors,” AMB Express 9, 70 (2019).
- www.nytimes.com/2017/11/27/health/gene-therapy-virus-shortage.html.
- A.J. Valkama, et al., “Optimization of Lentiviral Vector Production for Scale-Up in Fixed-Bed Bioreactor,” Gene Ther. 25(1), 39–46 (2018).